A (Very) Close Look at Carbon Capture and Storage - 6 minutes read
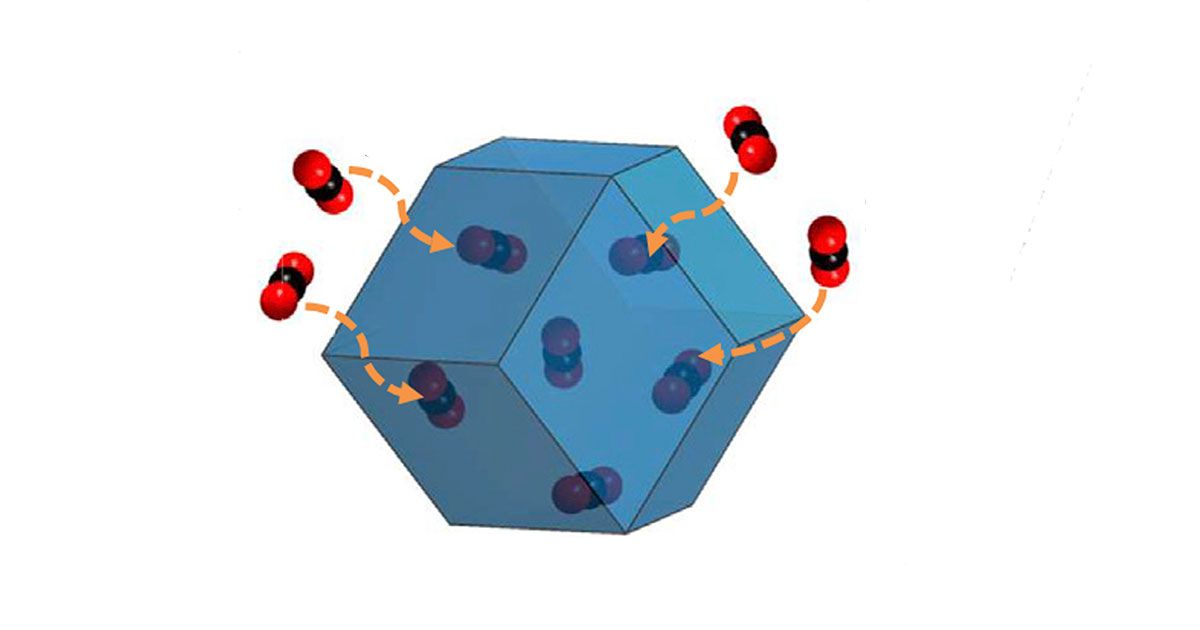
A new kind of molecular-scale microscope has been trained for the first time on a promising wonder material for carbon capture and storage. The results, researchers say, suggest a few tweaks to this material could further enhance its ability to scrub greenhouse gases from emissions produced by traditional power plants.
The announcement comes in the wake of a separate study concerning carbon capture published in the journal Nature.The researchers involved in that study found that keeping the average global temperature change to below 1.5 degrees C (the goal of the Paris climate accords) may require more aggressive action than previously anticipated. It will not be enough, they calculated, to stop building new greenhouse-gas-emitting power stations and allow existing plants to age out of existence. Some existing plants will also need to be shuttered or retrofitted with carbon capture and sequestration technology.
The wonder material that could potentially help is a cage-like lattice inside which individual carbon dioxide (CO) molecules can be trapped. Called a metal-organic framework (MOF), the material (consisting of metal ions attached like K’NEX hubs to rods made of organic molecules) also holds promise as a medium for drug therapies, desalination filters, nuclear waste containers, and photovoltaics.
Researchers are exploring MOFs because the materials can envelop many carbon dioxide molecules at once. The reason has to do with their remarkable sponge-like quality of containing holes and hollows nearly anywhere in the molecule one cares to look. Unfold all the caverns contained within an MOF into a single sheet, and just one gram of the stuff contains enough molecular surface area to occupy two football fields. Which can translate to a lot of holding space for CO—or many other types of molecules.
However, a CO molecule venturing into a MOF is like a mouse entering a Rube Goldberg mousetrap. It’s a complex and delicate process—so much so that the interaction had previously only been the subject of educated guesswork, and never directly observed.
Now, a new bio-imaging technology (itself the subject of a 2017 Nobel Prize) has captured the world’s first images of COmolecules caged inside a MOF. The images, the researchers say, provide the first glimpse into the ways carbon dioxide wanders into the cage and how that same trap can be made bigger and more absorbent. Further imaging and study, they say, would enable researchers to engineer, as it were, a better and more effective CO2 “mousetrap.”
The images were taken at SLAC National Accelerator Laboratory, home to a leading cryogenic electron microscope (cryo-EM) facility. This refrigerator-sized microscope probes flash-frozen samples using low-intensity electron beams and smart algorithms to produce images despite the comparative lack of electron “illumination” on the sample. Previous to cryo-EM imaging, some molecules and interactions went unobserved because harsh conventional electron microscope beams dissolved, melted, or otherwise harmed the sample. Biomolecules like blood platelets and cell receptors cannot be imaged nearly as reliably at the atomic scale as they can with cryo-EM.
In their new work, Yuzhang Li, a postdoctoral research fellow at Stanford University, and his co-authors used cryo-EM imaging to photograph the interaction of COwith a metal-organic framework molecule called ZIF-8. “We’re able to [image] the CO gas molecule and the metal-organic framework, stabilizing this interaction and the framework for the first time,” he says.
As noted in their paper, recently published by the journal Matter,the group found that the ZIF-8 molecule responded strongly to the presence of a CO molecule. The ZIF-8 framework, according to their imaging, expanded in size by 3 percent when it contained CO.
That may not seem like much. However, remember this is an individual, tiny carbon dioxide molecule that’s deforming the cage of the much larger ZIF-8 superstructure. The finding suggests, Li says, that ZIF-8 does indeed appear particularly responsive to CO—and is thus a good candidate for a carbon capture material.
“This observation implies a strong interaction between CO and the ZIF-8 framework, which further suggests that ZIF-8 might be a promising carbon capture material,” the researchers conclude.
Using cryo-EM for imaging biological samples is becoming commonplace today. Thousands of papers now reveal cryo-EM-derived structures of viruses, ribosomes, yeasts, receptors, and other biomolecules.
However, Li says using cryo-EM for non-biological materials remains more of a novel application. In 2017, he and collaborators imaged a dendrite from a lithium ion battery using cryo-EM. And Li says cryo-EM could not only bolster the search for molecules to aid in carbon capture, but that his group could also image dendrites in next-generation materials to assist in battery research and development.
“Similar to MOFs, high-energy battery materials are extremely beam sensitive, and sensitive to the environment—if you expose them to air, they’re going to corrode very quickly,” he says. “[Our] cryo-EM protocol enables us to stabilize battery materials [too]. It seems like a natural extension.”
Source: Ieee.org
Powered by NewsAPI.org
Keywords:
Carbon capture and storage • Molecule • Microscope • Materials science • Carbon capture and storage • Research • Raw material • Greenhouse gas • Air pollution • Power station • Research • Carbon capture and storage • Nature (journal) • Research • Research • Temperature record • Social change • Celsius • Paris • Climate • Action theory (philosophy) • Greenhouse gas • Power station • Ageing • Power station • Carbon capture and storage • Technology • Materials science • Crystal structure • Carbon dioxide • Carbon monoxide • Molecule • Metal-organic framework • Meta-Object Facility • Materials science • Metal • K'Nex • Organic compound • Desalination • Filtration • Radioactive waste • Photovoltaics • Materials science • Carbon dioxide • Sponge • Molecule • Football • Molecule • Mouse • Rube Goldberg • Mousetrap • Scientific method • Nobel Prize • Meta-Object Facility • Carbon dioxide • Carbon dioxide • Mousetrap • SLAC National Accelerator Laboratory • Cryogenics • Electron microscope • Cryo-electron microscopy • Refrigerator • Flash freezing • Cathode ray • Algorithm • Image • Lighting • Cryo-electron microscopy • Medical imaging • Molecule • Interaction • Electron microscope • Biomolecule • Platelet • Cryo-electron microscopy • Postdoctoral researcher • Stanford University • Cryo-electron microscopy • Medical imaging • Metal-organic framework • Molecule • Zero insertion force • Carbon monoxide • Gas • Molecule • Metal-organic framework • Scientific literature • Matter • Zero insertion force • Molecule • Carbon dioxide • Molecule • Zero insertion force • Medical imaging • Volume • Carbon dioxide • Carbon dioxide • Molecule • Zero insertion force • Base and superstructure • Zero insertion force • Carbon dioxide • Materialism • Observation • Strong interaction • Zero insertion force • Zero insertion force • Materials science • Research • Cryo-electron microscopy • Biology • Sample (material) • Cryo-electron microscopy • Cladistics • Biomolecular structure • Virus • Ribosome • Yeast • Receptor (biochemistry) • Biomolecule • Cryo-electron microscopy • Dendrite • Lithium-ion battery • Cryo-electron microscopy • Cryo-electron microscopy • Molecule • Carbon capture and storage • Dendrite • Materials science • Battery (electricity) • Research and development • Particle physics • Battery (electricity) • Materials science • Beam (structure) • Atmosphere of Earth • Cryo-electron microscopy • Battery (electricity) • Materials science •