Quantum Physics Isn't as Weird as You Think. It's Weirder - 6 minutes read
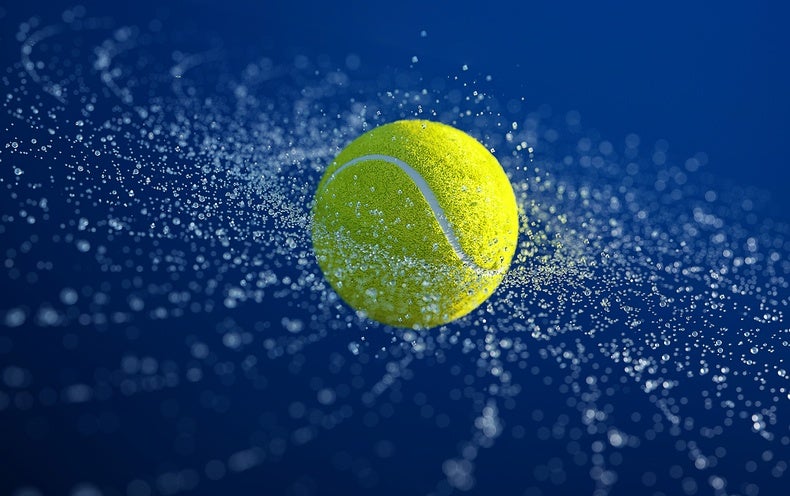
Down at the level of atoms and electrons, quantum physics describes the behavior of the very smallest objects. Solar panels, LED lights, your mobile phone and MRI scanners in hospitals: all of these rely on quantum behavior. It is one of the best-tested theories of physics, and we use it all the time.
On the face of it, however, the quantum realm is extraordinary: Within it, quantum objects can be “in two places at once”; they can move through barriers; and share a connection no matter how far apart they are. Compared to what you would expect of, say, a tennis ball, their properties are certainly weird and counterintuitive.
But don’t let this scare you off! Much of quantum physics’ odd behavior becomes a lot less surprising if you stop thinking of atoms and electrons as minuscule tennis balls, and instead imagine any “quantum object” as something like a wave you create by pushing your hand through water. You could say that, at small scales, everything is made of waves.
In the spirit of demystifying quantum behavior, here are three key types of “weird” quantum phenomena that normal water waves can do just as well, and the one thing that sets the quantum world apart.
NOT WEIRD: HEISENBERG’S UNCERTAINTY PRINCIPLE
Imagine throwing a tennis ball. If we wanted to, we could track the ball’s exact position and velocity throughout its flight. Strangely enough, if we were to shrink the ball down to the size of, say, an atom, this kind of tracking becomes impossible.
This limitation is called Heisenberg’s uncertainty principle. In quantum physics, it is impossible to know an object’s precise position and momentum (its velocity times its mass) at the same time. A tennis ball’s momentum is just its mass multiplied by its velocity, but for waves we determine momentum by measuring the distance between successive wave crests, a factor called the wavelength.
Waves are fickle, however, making it impossible to determine their position and wavelengths with 100 percent precision. In practice, any wave, whether watery or quantum, will always cover a range of positions, and consist of a range of wavelengths. The more you restrict one of those ranges, the less you can control the other.
Consider two extreme types of water waves: The first is an infinitely repeating wave of regularly spaced ripples made by the wind on an endlessly long canal. There you could measure the wavelength by identifying the repeating pattern of wave crests and troughs. But you can’t say anything about the wave’s “position” in the canal because it doesn’t have a start or end point. Conversely, for a wave consisting of a single, thin crest in an otherwise calm pond, you can measure its position, but it doesn’t have a well-defined wavelength because it never repeats.
In practice, all waves lie somewhere in between these two limits. Quantum waves are no different.
NOT WEIRD: SUPERPOSITIONS AND ENTANGLEMENT
A quantum object can “be in two places at once” by being in a so-called superposition of states. Thinking about waves, this is no surprise. A wave can be in two places at once. If you send a wave down a forked channel, it will easily split and flow through both channels at the same time.
A related quantum concept is entanglement, which combines superpositions in two waves. In a salad dressing that has been left to stand, for example, oil will float on top of the vinegar. Carefully making a wave in the oil will then also cause a wave in the vinegar, which looks like ripples in their interface. Measuring the wavelength of the oil wave also tells us about the wavelength of the vinegar wave. In other words: the two waves are linked, and their properties depend on one another.
Pouring the separated salad dressing down a forked channel, this remains true, so that the combined oil-vinegar ripples move down two channels at the same time. Measuring the wavelength of just the oil wave in one channel, you immediately know all wavelengths in both channels, even if they are far apart. Had the salad dressing been quantum, you would say that the waves in the two channels are “entangled” with one another. Quantum technology uses entanglement to create unbreakable encryption or speed up computations. For your salad, breaking the entanglement by shaking the dressing into a vinaigrette is probably more useful.
NOT WEIRD: TUNNELING
Another seemingly peculiar feat of quantum objects is that with some probability they can pass through barriers. This is called tunneling. Throw a tennis ball at a wall and (as long as the wall remains standing) it will bounce back. Do this with an atom, and you might find it on the other side.
In some cases, a water wave can move through a barrier just like a quantum particle, something you can demonstrate in your bathtub. To do so, build an underwater wall in the tub, one tall enough that it almost touches the water’s surface, but not quite. If you send a wave at this wall at a glancing angle, it will always bounce back from the wall. This is analogous to so-called total internal reflection of light rays. It depends only on the height of the barrier and the angle with which the wave approaches the wall.
Although the wave cannot travel over the barrier, a small tail of it can probe the other side. If the wall is thin enough, you will see the tail remembering its original motion and magically reappearing as a traveling wave. Voilà, your water wave has tunneled through a wall! The same phenomenon of “broken” total internal reflection, but with light rays instead of water waves, is used in certain types of touch screen displays.
VERY WEIRD: QUANTUM MEASUREMENT
Whereas most weird quantum behaviors are demystified by thinking of small particles as waves instead of minuscule balls, genuine quantum weirdness arises when you measure a quantum object. Whether it’s a wave traveling through two different channels, or one that’s tunneled through a barrier, measuring a quantum wave results in the entirety of that wave suddenly appearing in a single location: in one channel and not the other, or on one side of the barrier and not the other. This doesn’t happen with salad dressing.
Funnily enough, the mathematical equations that describe quantum waves do not explain what happens when we measure them. Physicists don’t yet agree on how best to describe or interpret this process. Quantum measurement is the one thing that sets quantum behavior apart from water waves, truly making quantum physics strange.
To appreciate how unusual quantum measurement is, imagine someone speaking to a crowd of people. Sound waves spread out across the crowd, and everyone hears the speech. In the quantum world, however, the sound wave would spread out just as expected, but as soon as a single person in the crowd perceives (or measures) it, the entire sound wave would concentrate itself in that single person’s ear, and no one else would hear it.
Now that is weird.
This is an opinion and analysis article, and the views expressed by the author or authors are not necessarily those of Scientific American.
Source: Scientific American
Powered by NewsAPI.org